Here's Everything You Need to Know About the Life Cycle of Stars
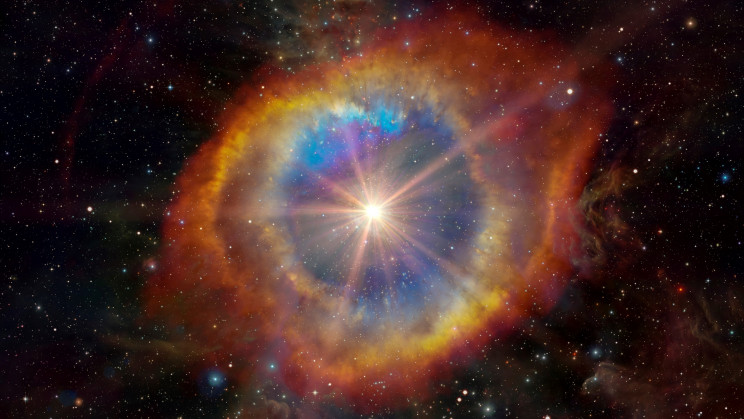
Stars are giant balls of gas that emit light and heat. They are mostly made up of hydrogen and helium gases and can have huge masses. For instance, the heaviest star yet found in our universe, called R136a1, has a mass of around 315 times that of our Sun and is almost 9 million times more luminous.
Stars are so heavy that they should collapse due to the inward force of gravity exerted by their own weight but thanks to the nuclear fusion reactions taking place in their cores, the massive inward gravitational force is balanced by the strong heat and pressures found within a star. This balance between gravity and gas pressure from energy generation is called hydrostatic equilibrium, and it is both self-regulating and finely tuned.
So if the rate of energy generation in the core reduces, then gravity becomes more prominent, and the star will begin to contract, increasing the temperature and pressure of the star’s core, which can lead to higher energy generation and a return to equilibrium. However, that does not imply that stars are eternal. Just like us, they also go through a cycle of birth and death.
How a star is born?
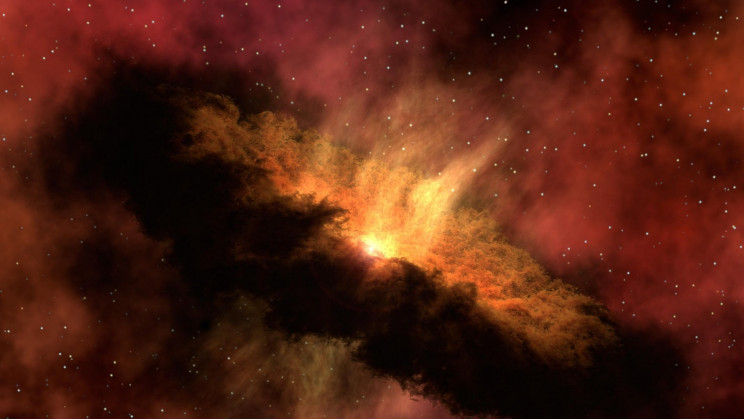
The interstellar medium (the region between the stars) contains many giant, diffuse clouds of dust and gas (mostly hydrogen and helium). These clouds are called nebulae, and they generally cover areas that span across many light-years. Some well-known nebulae include the Eagle Nebula (which contains the Pillars of Creation), the Coal Sack Nebula (a dark nebula visible to the naked eye), and the Orion Nebula (which is also visible to the naked eye).
Although the gas and dust in a nebula are spread out, eventually, turbulence within the nebula causes clumps called knots to begin forming, and attract more and more gas and dust owing to gravity. As the mass of this clump reaches a sufficient value, it starts collapsing under its own gravitational force. The matter inside the knot becomes denser and denser, and the temperature of the matter at the center of the knot increases.
This hot and dense core becomes a protostar. With enough matter present, the collapse will continue, and the core of the protostar will continue to become denser and hotter. Eventually, it will become hot and dense enough for hydrogen to fuse into helium, releasing large amounts of heat and light. It is at this point that a star is born!
The main stage when a star becomes mature
This process of the cosmic dust cloud collapsing and eventually forming a star can take millions or billions of years. It is also important to note that not all of the material in the clump will become part of the star; the leftover material can turn into planets, asteroids, or comets, or even remain as dust.
Once a star is formed and fusion starts inside its core, it doesn’t continue to collapse because the energy expelled from the nuclear fusion reaction in its core causes an outward radiation pressure which counter-balances the inward gravitational pull, keeping it in an equilibrium state. The stage at which the thermonuclear fusion stabilizes is the mature phase of the star and is referred to as the main sequence.
Though the process has been simplified here, stellar fusion is not a simple fusion of two hydrogen atoms to form helium. At the high pressures and temperatures of the star’s core, the electrons separate from the nuclei, leaving behind plasma, a mix of positively charged ions and electrons.
When two hydrogen ions, which are just protons, collide, they form the nucleus of an isotope of hydrogen called Deuterium and positrons. And when two such Deuterium nuclei merge, they form a helium nuclei (He-4). Or, they can interact with other protons to make another isotope of helium (He-3). Two He-3 nuclei can then fuse to make an unstable beryllium nucleus (Be-6), which breaks apart to give He-4 and two protons. Energy is released at each step.

The star’s energy output, color, temperature, brightness, and lifespan vary according to the amount of matter involved during its birth. A star’s color hints at its temperature and size (refer to the Hertzsprung Russel Diagram above) - the red ones are the coolest and smallest, and the blue ones are the hottest and largest.
Death of a star
The fusion of hydrogen ions to form helium nuclei is basically what fuels the bright shining lifecycle of a star. However, a star has a limited amount of hydrogen in its core. Once all the hydrogen in the core is fused, the nuclear reactions no longer take place and the star starts collapsing again under the force of its gravity.
Meanwhile, the excess hydrogen available outside the core forms a shell, and fusion continues in this shell. This results in a situation where the core is contracting due to gravity and getting denser and hotter, and the shell is expanding due to fusion and cooling down. This larger, late-stage star is called a “Red Giant".
The hotter core may also support other nuclear reactions that use helium to form heavier elements, but these reactions release less and less energy and cannot sustain the Red Giant. These atomic reactions eventually build an outward pressure that pushes the outer layers of the star farther out. The size of the star determines the eventual fate of the Red Giant stage.
In low or medium-sized stars (up to about 7 times the mass of the Sun), once the helium in the core is gone, the star will shed most of its mass, forming a cloud of material called a planetary nebula. The core will continue to cool and shrink, leaving behind a small, dense ball called a white dwarf. The pressure of electrons repelling each other in its core prevents the white dwarf from collapsing further.
However, as the fusion reactions slow and stop, the white dwarf will eventually cool down until it reaches the same temperature as its surrounding environment. At this point, it is called a black dwarf.
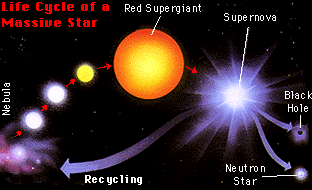
In a binary or multiple star system, if a white dwarf is close enough to another star, then it can suck some of the hydrogens from the other star’s outer layers onto its own surface. This hydrogen again starts to fuse and expels the remaining material. This process can repeat itself. Whenever fusion starts again, there will be a sudden increase in brightness and then a slow return to its original state, hence they are termed nova.
In larger stars, the process is similar — when the supply of helium runs out, the core will contract. However, if the core has enough mass, other fusion reactions occur, until the core is filled with iron atoms. Up to this point, the energy output allows the star to fight the inward force of gravity. However, fusing iron to form heavier elements requires a lot of energy, so once enough iron is present, the star no longer outputs enough energy to maintain equilibrium and loses the fight against gravity.
As gravity pushes the iron atoms closer together, the core shrinks to a very small size (a few miles across), and temperatures rise tremendously. Eventually, the repulsive force between the positively-charged nuclei overcomes the force of gravity, and the core expands violently in the shockwave of a supernova.
In a supernova, around 75% of a star's mass is ejected into space. Eventually, the dust and gas from the supernova will come together due to gravity, turn into a nebula, and the cycle continues.
Post a Comment