What are Neutron Stars? All About These Enigmatic Stellar Corpses
When a star dies, there are a few stellar objects that can be left behind, from the relatively mundane white dwarf to the enigmatic black hole. But in between these two extremes lies the neutron star, an object of such immense density that few other objects in the universe even come close.
Unlike its denser cousin, the black hole, neutron stars are visible and highly active in ways that few other objects in the universe are, making them fascinating objects of study for astronomers.
What are neutron stars though? How do they form? How are they different than black holes? And could the Sun become a neutron star? Read on to learn this, and more, about these incredible celestial objects.
What is a Neutron Star?
When a star exhausts its fuel, one of several things can happen as a consequence of its mass.
If the star is small enough, it will likely form a white dwarf. If it is massive enough, it will end its life as a black hole. Neutron stars occupy the space in between these two outcomes, though they are by no means the typical fate of a main sequence star.
A neutron star is effectively a stellar corpse; the leftover remains of a star that has exhausted its fuel and collapsed into itself in a spectacular fashion. It no longer burns hydrogen, helium, or any other element as fuel, and so is for all intents and purposes dead matter.
That doesn't mean that it is cold and inactive, however.
A neutron star is often the remnants of the core of a star big enough that when its mass collapses after nuclear fusion ends, it is so dense that the vast majority of the electrons and protons of the atomic nuclei in its core fuse together to form neutrons.
This produces all kinds of incredible phenomena that we are only just beginning to understand.
Are Neutron Stars Rare?
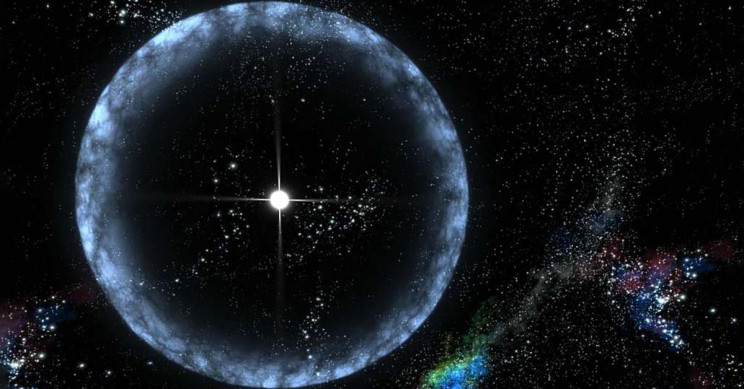
Relatively speaking, yes. A very specific condition has to apply for a neutron star to form, and it really all comes down to the mass of the star.
When high-mass stars exhaust all of their fuel, they reach a point where they start to fuse iron, but that fusion no longer releases energy as a result. Without the outward force of fusion energy in their cores to counter the effect of gravity and hold up their incredible mass, these stars undergo gravitational collapse.
If a star is greater than about eight solar masses but less than 20 to 30 solar masses, its core will be incredibly condensed, but not so much that the force of the condensed material pushing outward is unable to keep the star from completely collapsing under its own weight towards infinite density.
There aren't a whole lot of stars in the galaxy that satisfy this tight weight condition, but astronomers think that there are about one billion neutron stars in the Milky Way, which is about equal to the number of stellar-mass black holes in the galaxy.
While one billion sounds like a lot, compared to the estimated 400 billion stars in the Milky Way, one billion neutron stars would be about 0.25% of all "stars" in the galaxy. So yes, neutron stars are rare.
How Do Neutron Stars Form?
Neutron stars form from the gravitational collapse of an especially large star, between eight to 30 solar masses.
Counter-intuitively, the larger the star, the quicker it burns through its fuel since the bigger the star, the greater the amount of surface area from which it can radiate away energy. So, a star that is 30 times the mass of our Sun will burn through its hydrogen fuel in a fraction of the time it would take our Sun to do the same.
The larger star's red giant phase, during which it primarily fuses helium, will finish even quicker than the billion years it will take our Sun to exhaust its helium reserves.
The subsequent fusion of oxygen, carbon, and silicon into iron will happen even faster, and this is the upper limit of elements that any star can fuse together for energy.
By now, the outward force of whatever fusion is taking place can no longer balance out the gravity of so much mass, so at this point, the star rapidly collapses into its core. It does so with such violence that it presses the core into an impermeable substance whose density is comparable to a sugar cube-sized ingot with the mass of Mt. Everest.
Understandably, the star's outer material simply bounces off the core like a racquetball hitting a concrete wall off an opening serve. This produces a sudden—and spectacular—explosion as all of that energy is directed outward into space instead, the result of which we call a supernova. These can be so energetic and luminous that we can see them even in distant galaxies.
The remaining lump of the star's core, meanwhile, remains smushed together into a super-dense ball from the violent forces of the star's gravitational collapse, and is now what we would call a neutron star.
How Big is a Neutron Star?
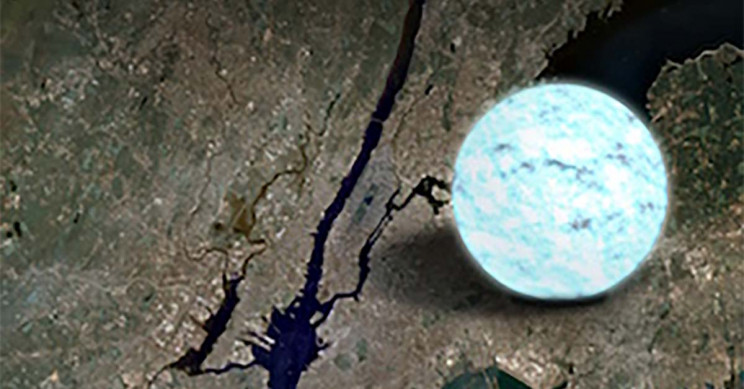
Big is not at all the right word to use here. A neutron star is incredibly tiny by astronomical standards. In fact, there are plenty of asteroids in the solar system that are many times larger than a typical neutron star.
An average neutron star is about 12 miles in diameter (19.3 km), which is roughly within the distance between the easternmost edge of New York City (starting at the border of the borough of Queens with Nassau Country, Long Island) and midtown Manhattan. In fact, it wouldn't even stretch as far as downtown Brooklyn and might barely reach as far as the Williamsburg Bridge.
Essentially, a neutron star is about a fifth of the size of America's greatest city—less than half the distance a runner would have to cover in the New York City marathon—but still packs in as much as three times the mass of the Sun.
Keep in mind that the mass of the Sun is capable of keeping the Kuiper Belt, including Pluto, in orbit, even though it is billions of miles away. Now imagine something two and a half to three times as massive, all pressed into a volume the diameter of which couldn't even cover the length of one of New York City's outer boroughs. That's how dense a neutron star is, and it results in some really crazy physics.
Which Stars Become Neutron Stars?
Neutron stars can only form from a specific type of star. If the original star is less than about eight solar masses, then once it burns through its helium fuel, the core of the star will "shed" its outer layer of material.
This leaves behind a dense core of material known as a white dwarf, surrounded by a so-called planetary nebula (no planets are actually involved in the creation of this nebula, early astronomers just confused these nebulae with planet-forming processes, and the name just stuck).
If the leftover material is heavier than roughly about three solar masses, then not even the physical repulsion of the strong nuclear force that binds quarks together into protons, electrons, and neutrons will be enough to hold back gravitational collapse, and a stellar-mass black hole will form.
If the material is in this estimated range between 1.4 and about 2.2 solar masses, then the electrons of most of its atoms get squeezed up against the corresponding atomic protons and merge to create a dense mass of neutrons.
The neutrons still have enough physical presence and force to prevent the utter collapse of the star, however. While the neutrons of the neutron star might be as physically compact and dense as is physically possible, they are able to push back just enough against gravity that the inward collapse doesn't continue towards infinity.
What Are the Types of Neutron Stars?
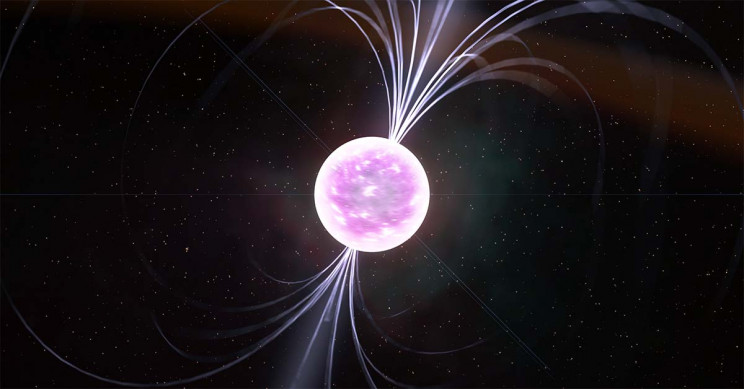
Not surprisingly, neutron stars are rather diverse; after all, when you are brushing up against a singularity-inducing density, things are bound to be complicated.
So far, we have identified as many as nine different neutron star types—each as exotic as the next.
Radio pulsars are a type of neutron star that are highly magnetized and which rotate on their axis very rapidly, as often as dozens of times in a single second, and are characterized by a relatively tight beam of radiation that replicates a lighthouse's light beam (only much, much faster).
Recycled Pulsars are similar to regular radio pulsars but are part of a binary system and are theorized to have lost energy over time and had begun to fade out, but have been reinvigorated by material accreted from its partner star.
A Magnetar is an object that doesn't seem like it should exist. Neutron stars are made of neutrons, after all, so how can they possibly produce a magnetic field? Well, there are some protons still stuck inside those neutron stars and they are being compressed under incredible pressures. This is the kind of environment that produces physics that can only be described as weird.
How weird? Well, the Earth's magnetic field can be measured in gauss units. The Earth's magnetic field is about one gauss unit, while an MRI is about 10,000 gauss units. A magnetar? Its magnetic field is measurable in the trillions of gauss units, and even into the quadrillion gauss unit range—despite being a fraction of Earth's diameter.
This is powerful enough that getting within 620 miles (1,000 km) of a magnetar would be close enough that the magnetic field of the magnetar would just uncouple electrons from the protons in your body and rip apart any chemical bonds between the elements that make up the proteins that make up our bodies.
A magnetar's magnetic field is so powerful, then, that we would suddenly disintegrate into subatomic particles if we got within 620 miles (1,000 km) of one.
A Soft Gamma Repeater is a type of magnetar that is characterized by outbursts of gamma and X-ray radiation. They are theorized to occur when the magnetic field of the magnetar shifts and fractures the crust of the neutron star in a process known as a starquake. During such an event, electrons and positrons are ejected at high speeds and their interaction generates the radiation we observe.
These outbursts can have a huge impact on our environment, though they do little to harm us here on the ground. Still, in 1998, an outburst from SGR 1900+14 was sufficient to ionize Earth's upper atmosphere and interfere with radio communication, despite originating around 20,000 light-years from Earth.
An Anomalous X-ray pulsar is likely a magnetar whose pulse period is too slow to power X-ray emission, but produces them anyway. The prevailing theory is that the X-rays from an Anomalous X-ray Pulser are created as the magnetic field of the magnetar decays.
Low-mass X-ray binaries are binary star systems with either a black hole or a neutron star along with a relatively dim late-stage star. The neutron star or black hole accretes material from its companion and forms it into a disk around itself.
The material in this disk is moving at relativistic speeds and effectively rubbing up against neighboring material, generating lots of X-rays in the process.
Intermediate-mass X-ray binaries are also binary star systems with a black hole or neutron star sucking away material from a companion star, though in this case the companion is an intermediate-mass star larger than our Sun.
Eventually, enough material is siphoned away by the neutron star or black hole that it is reclassified as a low-mass X-ray binary.
As you can guess, a high-mass X-ray binary is also a binary star system with a neutron star or black hole, though its companion is an especially large star, like a blue supergiant or a Wolf-Rayet star. In high-mass X-ray binaries, the larger star tends to dominate the electromagnetic spectrum in visible light, while the smaller, neutron star/black hole produces a large amount of X-ray radiation.
Finally, there are the accretion-powered pulsars. According to NASA, these are X-ray binary pulsars "whose pulses are generated by the accretion flow striking the neutron star. Instead of falling uniformly onto the neutron star, the steady flow (accretion) of matter from the companion star is channeled by the pulsar's magnetic field onto the magnetic poles of the neutron star, resulting in a pair of "hot spots" on the pulsar surface."
What Would a Pulsar Look Like?
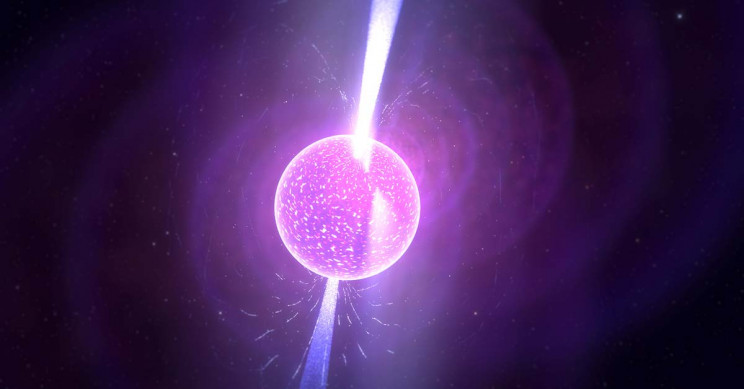
It depends on how you are looking at it and with what instrument. If you were to look at it with the naked eye, it would likely look like a white dwarf, slowly radiating away its heat for billions of years.
Look at it using a radio telescope, however, and you see things much differently. Pulsars emit a tight beam of radio waves, which humans cannot see. Our radio telescopes can, however, and to them, pulsars look something like a lighthouse sweeping its light over a darkened sea.
This sweep happens incredibly fast though. Because neutron stars conserve their spin from when they were much physically bigger stars, while preserving their mass, they spin incredibly fast.
This means that the tight beam of radio waves can spin around a hundred times a second or even faster, producing the pulses of radio waves that give a pulsar its name.
What Does Neutron Star Size Reveal about Them?
The size of a neutron star tells us a couple of things.
First, it can tell us about the type of star that originally formed it. For the most part, a star's mass is preserved throughout its lifetime, So once we get a sense of how massive a neutron star is, we can extrapolate this data to how big the star that formed it originally was.
Moreover, a neutron star's size can tell us about the likelihood that it will eventually become a black hole. If two neutron stars collide, their mass is combined and it is possible that the merger can result in either a much bigger neutron star or a stellar-mass black hole.
We don't quite know what the upper limit of mass is for a neutron star, so observing neutron stars that collide can tell us much about how black holes are able to form and under what conditions.
This could hopefully give us a better sense of how black holes might form in the universe beyond the standard stellar collapse model of black hole formation, as well as give us key insight into the densities of these exotic celestial objects.
Is a Neutron Star a Black Hole?
No, in a nutshell, a neutron star is not a black hole, but it comes about as close as anything else in the universe can to becoming one.
Precise measurements need to be refined to narrow down the range of stars that collapses into neutron stars and which ones become black holes, and there is some evidence from neutrino bursts that a star can first collapse into a neutron star and then collapse again into a black hole, so a neutron star might not be the final state of a star.
It is important to distinguish between a neutron star and a black hole, though, since they are very near to one another, but also an infinite distance apart.
Can a Neutron Star Become a Black Hole?
Under some circumstances, yes—though not all neutron stars will do so.
Since mass is the be-all and end-all of black hole formation, neutron stars are the only objects that really have a chance to evolve into black holes outside of initial stellar collapse.
If a neutron star is part of a binary star system, it can start accreting material from its companion star and build up its considerable mass even further.
When this happens, the mass of the neutron star can mathematically cross a point where escape velocity—that is, the speed that something needs to be moving in order to break free of an object's gravity—exceeds the speed of light itself.
When this happens, the neutron star becomes a black hole, and no light can escape its event horizon, shrouding its interior in darkness forever. Since no light or any other data can escape its interior, we literally can't say what happens next. Although some physicists have demonstrated that black holes may actually be able to shed information.
Whether the neutron star remains as it is or whether it collapses even further into infinity is an open question that will likely never be satisfactorily answered.
What Happens When Two Neutron Stars Collide?
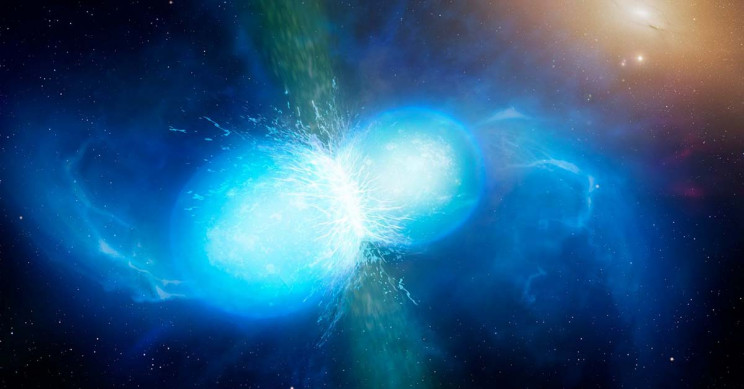
Objects collide in space all the time, whether it's planets, stars, or even whole galaxies. Neutron star collisions are pretty special though, for a number of reasons.
First, when neutron stars collide, they will always merge into a single object due to their intense gravitational fields, whereas the collisions of some other types of stars can destroy the stars and leave behind vast nebulae of hydrogen gas.
These mergers can produce a magnetic field trillions of times more powerful than Earth's in a matter of milliseconds, producing short gamma-ray bursts that are some of the most powerful bursts of energy seen in the universe since the Big Bang.
These kinds of mergers are also important, since they may be the source of much of the heavier elements in the universe, like gold, which are produced during a process known as rapid neutron capture.
Since the atomic nuclei of elements heavier than iron can quickly decay, they need neutrons to help stabilize them and they need them very fast. Neutron star mergers are thought to be one of the few places where such rapid neutron capture is possible. It isn't the only way this may happen though, so there is still a debate about whether this is the case.
Neutron star mergers are also one of the few events capable of generating gravitational waves (the other two being neutron star-black hole mergers, and two black holes merging), and so they are incredibly powerful events capable of literally shaking the fabric of space-time.
Will the Sun Become a Neutron Star?
In short, no, it will not.
Astronomers have studied the Sun for literally millennia. We know its mass, more or less, and we can reasonably predict its fate several billion years into the future.
The Sun simply doesn't have the mass to form a neutron star. When the Sun exhausts its hydrogen fuel, it will expand rapidly into a helium-fusing red giant. When it exhausts that fuel, that's pretty much it.
It just isn't massive enough to fuse that helium any further, especially in its outer layers, so it will simply let go of all that extra bulk like a cicada shedding its exoskeleton.
This excess material will go on to form a planetary nebula around the white-hot remnant core of the Sun, which would now be classified as a white dwarf. In the end, our Sun is destined to look more like the standard nebula than anything else.
Post a Comment